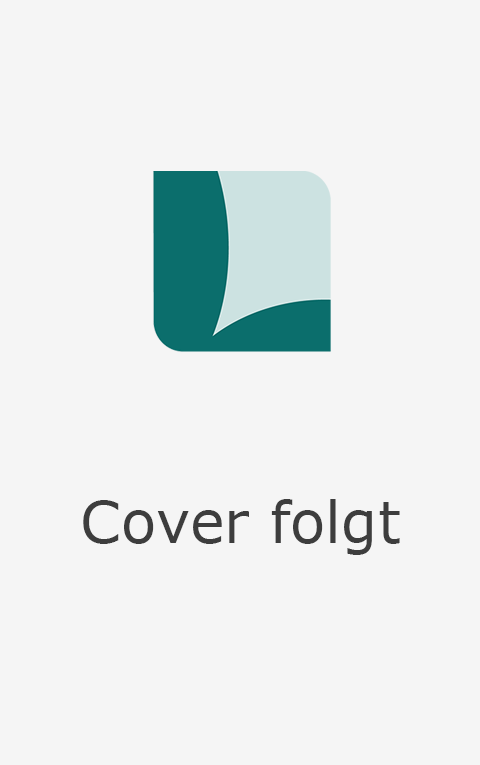
Model Organisms in Spinal Cord Regeneration
Wiley-VCH Verlag GmbH (Hersteller)
978-3-527-61036-5 (ISBN)
- Keine Verlagsinformationen verfügbar
- Artikel merken
This handbook provides a comprehensive overview for students, clinicians and researchers planning to enter the field of neural regeneration, combining the latest knowledge with an understanding of all important model organisms in one handy volume. By covering the strengths and weaknesses as well as possible applications of different models, it saves researchers both time and resources in their choice of the appropriate model organism. It is an equally valuable introduction for the novice planning to enter the field.
Catherina G. Becker is a senior lecturer and Thomas Becker is a senior researcher at the University of Edinburgh, UK. They are collaborating on spinal cord regeneration in adult zebrafish. Throughout their careers, the editors have shared a keen interest in central nervous system regeneration in different model organisms. They began their work in 1993, when both received their PhDs form the University of Bremen, Germany, for the analysis of central nervous system development and regeneration in amphibians. Catherina and Thomas Becker continued their studies, focusing on the role of cell recognition molecules in zebrafish, during postdoctoral studies at the Swiss Federal Institute of Technology in Zurich, Switzerland, the University of California at Irvine, USA, and at the University Hospital in Hamburg, Germany. From here they moved their group to Edinburgh. Their research has been continually supported by prestigious national and international fellowships and research grants.
Preface.List of Contributors.I Mammalian Models of CNS Regeneration.1 The Role of Inhibitory Molecules in Limiting Axonal Regeneration in the Mammalian Spinal Cord (Patrick N. Anderson, Jez Fabes, and David Hunt).1.1 Introduction.1.1.1 CNS Neurons Have Widely Differing Phenotypes.1.2 Difficulties in Assessing Axonal Regeneration in the Mammalian Spinal Cord.1.2.1 Experimental Lesions and Problems of Interpretation.1.2.2 Tracing Regenerating Axons.1.2.2.1 Regeneration of Corticospinal Axons is Difficult to Assess.1.2.2.2 Regeneration of Ascending Dorsal Column Axons Can Be Measured Simply and Accurately.1.3 Myelin Proteins as Inhibitors of Axonal Regeneration.1.3.1 Nogo.1.3.2 OMgp.1.3.3 MAG.1.3.4 The Nogo-66 Receptor, NgR1, (RTN4R), and Related Molecules.1.3.5 Co-Receptors: LINGO-1, p75 and TROY (TAJ).1.3.6 Signal Transduction from Myelin-Derived Inhibitory Molecules.1.3.7 The Role of Nogo-A in Axonal Regeneration in the Spinal Cord.1.3.7.1 Variations in the Extent of Axonal Regeneration in Different Strains of Nogo Knockout Mice.1.3.7.2 Effects of Antibodies Against Nogo on Axonal Regeneration in Spinal Cord.1.3.7.3 Neuronal Nogo-A.1.3.8 The Role of NgR1, NgR2 and Their Co-Receptors in Axonal Regeneration Within the Spinal Cord.1.3.8.1 The Distribution of NgR1 and NgR2 Does Not Suggest a General Regeneration-Inhibitory Function in the CNS.1.3.8.2 Knockout Mice Do Not Provide a Clear Picture of the Role of NgR1 in Regeneration.1.3.8.3 Pharmacological Blockade of NgR1 Enhances Axonal Sprouting and Regeneration.1.3.8.4 The Pattern of Expression of LINGO-1 and p75 Does Not Suggest a General Role in Inhibiting Regeneration in Vivo.1.3.8.5 LINGO-1, p75 and TROY Have Important Roles in Neurite Outgrowth in Vitro, But Their Significance for Axonal Regeneration in Vivo Has Not Yet Been Established.1.3.9 Effects of MAG and OMgp on Axon Regeneration in the Mammalian CNS.1.3.10 Strong Evidence That Myelin Proteins Are Not Always Effective Inhibitors of Axonal Regeneration in Vivo.1.4 Inhibitors at the Lesion Site.1.4.1 CSPGs.1.4.1.2 Relationship Between the Distribution of CSPGs and Failure of Axonal Regeneration.1.4.1.3 Chondroitinase ABC and Axonal Regeneration.1.4.1.4 Scar-Reducing and Growth-Promoting Effects of Decorin.1.4.2 Axonal Guidance Molecules Are Present in the Spinal Cord and Their Receptors Are Expressed by Specific Classes of Neuron.1.4.2.1 Semaphorins.1.4.2.2 Ephrins.1.4.2.3 Slits and Netrins in the Mammalian Spinal Cord.1.5 The Most Consistent Effects of Interfering with Inhibitory Molecules or Their Signaling Are on Raphespinal Axons.1.6 Interfering with Downstream Effectors of Inhibitory Signaling.1.6.1 Cyclic AMP Can Modulate the Responses of Neurons to Inhibitory Molecules in Vitro But is Only a Weak Promoter of Axonal Regeneration in the Spinal Cord.1.6.2 Rho-A Inhibition.1.6.3 Interfering with Other Targets on Signaling Pathways.1.7 Inhibitory Molecules and the Control of Neuronal Growth-Associated Genes.1.8 Conclusions.References.2 Intrinsic Factors Contributing to Axon Regeneration in the Mammalian Nervous System (Ferdinando Rossi).2.1 Introduction.2.2 Modes of Axon Growth.2.3 Developmental Regulation of the Intrinsic Neuronal Growth Properties.2.3.1 Purely Intrinsic Mechanisms.2.3.2 Target-Derived Factors.2.3.3 Factors Acting Along the Axon.2.3.4 Factors Acting on the Cell Body.2.4 Injury-Derived Regulation of Intrinsic Neuronal Growth Properties.2.4.1 Negative Regulation by Injury-Associated Factors.2.4.2 Positive Regulation by Injury-Associated Factors.2.5 Procedures to Enhance Intrinsic Growth Potential.2.5.1 Conditioning Lesions.2.5.2 Pharmacological Stimulation.2.5.3 Overexpression of Neuronal Growth Genes.2.6 How Does Up-Regulation of Neuronal Growth Genes Override Environmental Inhibition?2.7 Growth Control and Experience-Dependent Plasticity in the CNS.References.3 Stimulating Intrinsic Growth Potential in Mammalian Neurons (Bhavna Ylera and Frank Bradke).3.1 Introduction.3.2 Both Intrinsic and Environmental Factors Influence Axonal Growth.3.3 Intrinsic Growth Potential Depends on Neuronal Age.3.4 Signaling Pathways Involved in Influencing Intrinsic Regenerative Growth Potential.3.4.1 Role of cAMP in Axonal Regeneration.3.4.1.1 Therapeutic Strategies for CNS Repair Based on Stimulating Intrinsic Growth Potential of Neurons via the cAMP Pathway.3.4.2 Role of Conditioning Lesion in Axon Regeneration.3.4.2.1 The Conditioning Lesion Paradigm.3.4.3 Rho Signaling in Axon Regeneration.3.4.3.1 Role of PKC in Rho-Mediated Inhibition of Axon Growth.3.5 Identification of Genes and Proteins Involved in Axonal Regeneration.3.5.1 Genes Involved in Autodestruction and Neuroprotection.3.5.2 Cell Cycle Genes.3.5.3 Plasticity Genes.3.5.4 Spatiotemporal Alteration of Genes Involved in Cholesterol Metabolism, Apoptosis, Cell Cycle, and In.ammation.3.5.5 Late Changes in Gene Expression.3.6 Specific Proteins Involved in Axonal Regeneration.3.6.1 SPRR1A.3.6.2 Fibroblast Growth Factor-Inducible-14.3.6.3 GAP-43 and CAP-23.3.6.4 a7b1 Integrin.3.6.5 Retinoic Acid Receptor.3.7 Conclusions and Outlook.References.4 Cellular Grafting Strategies to Enhance Regeneration in the Mammalian Spinal Cord (Richard L. Benton, and Scott R. Whittemore).4.1 Introduction.4.2 CNS and Peripheral Nervous System (PNS) Tissue Transplantation Into the Injured Spinal Cord.4.3 Engineered PNS Tissue and Schwann Cell Transplantation in the Injured Spinal Cord.4.4 Adult/Postnatal and Fetal-Derived CNS Precursor/Sustentacular Cell Transplant-Mediated Regeneration in the Mammalian Spinal Cord.4.5 Non-CNS/PNS Cellular Grafting to Facilitate Host Axonal Regeneration.4.6 Summary and Perspective.References.II Mammalian and Non-Mammalian Models of Spinal Function.5 Spinal Motor Functions in Lamprey (Sten Grillner and Peter Wallen).5.1 Introduction.5.2 The Lamprey Model.5.3 Segmental Network: Basic Features.5.4 Cellular Properties of Importance for Operation of the Segmental Network.5.5 Modulator Systems: Action on Ion Channels Manifested on the Network-Behavioral Level.5.6 Segmental Network Modeling.5.7 Intersegmental Coordination: Biology and Mathematical Modeling.5.8 Movement-Related Feedback has Powerful Effects on the CPG.5.9 Comments on Sensory Coordination Across Complete Spinal Lesions: Lessons Learned from the Spinal Eel.5.10 Concluding Remarks.References.6 Genetic Approaches to Spinal Locomotor Function in Mammals (Anna Vallstedt and Klas Kullander).6.1 Introduction.6.1.1 The Spinal Cord Central Pattern Generator.6.1.2 Dissecting a Neuronal Network.6.2 Development of Spinal Cord Neurons.6.2.1 Origin of Ventral Spinal Cord Neurons.6.2.2 Function of Early Neuronal Classes.6.2.3 Creating Functional Diversity.6.3 Spinal Cord Neurons Involved in Locomotor Function.6.3.1 Location of the CPG.6.3.2 Motor Neurons.6.3.3 The Renshaw Cell.6.3.4 Interneurons Involved in Spinal Reflex Pathways.6.3.5 Excitatory Interneurons.6.3.6 Commissural Interneurons.6.4 Circuit Formation.6.4.1 Axon Guidance Molecules and Function of Neuronal Circuits.6.4.2 Neurotransmitter Phenotype During CPG Development.6.5 Emerging Technologies.6.5.1 Genetic Markers.6.5.2 Gene-Expression Initiatives.6.5.3 Creating Transgenics.6.5.4 The Combination of Cre-Expressing and Reporter Mice.6.5.5 The Principle of Reporter Mice.6.5.6 Visualization of Neurons.6.5.7 Visualization of Neuronal Connectivity.6.5.8 Visualization of Neuronal Activity.6.5.9 In-Vivo Imaging of Neuronal Populations.6.5.10 Functional Studies by Destruction of Neurons.6.5.11 Functional Studies Through Silencing of Neurotransmission.6.5.12 Functional Studies Through Activation of Neurons.6.6 Future Directions.References.7 Comparative Analysis of Descending Supraspinal Projections in Amphibians (Agustin Gonzalez and Hans J. ten Donkelaar).7.1 Introduction.7.2 The Pattern of Descending Pathways to the Spinal Cord in Amphibians.7.2.1 Anurans.7.2.2 Urodeles.7.2.3 Gymnophionans.7.2.4 Comparative Aspects of Brainstem-Spinal Descending Pathways.7.3 Development of Descending Pathways to the Spinal Cord in X. laevis.7.4 Chemically Characterized Descending Pathways.7.4.1 Catecholaminergic Pathways.7.4.2 Serotoninergic Pathways.7.4.3 Cholinergic Pathways.7.4.4 Nitrergic Pathways.7.4.5 Calbindin-D28k/Calretinin-Containing Pathways.7.5 Regeneration of Descending Supraspinal Pathways in Amphibians.References.III Non-Mammalian Models of Spinal Cord Regeneration.8 Regeneration in the Lamprey Spinal Cord (Michael I. Shifman, Li-Qing Jin, and Michael E. Selzer).8.1 Introduction.8.1.1 General Biology.8.1.2 Evolutionary Importance.8.1.3 Uses in Neuroscience Research.8.1.3.1 Neuroanatomy.8.1.3.2 Synaptic Transmission in the CNS.8.1.3.3 Pattern Generation.8.2 Axonal Regeneration.8.2.1 Background: Early Anatomic and Physiologic Studies.8.2.2 Demonstration of Functional Axon Regeneration.8.2.2.1 NIH Criteria.8.2.2.2 Evidence That the Experimental Lesion Disconnects Nerve Fibers.8.2.2.3 Anatomic Evidence That Regenerated Axons Bridge the Lesion and Make Junctional Contacts.8.2.2.4 Evidence That Regenerated Fibers Elicit Postjunctional Responses.8.2.2.5 Evidence That Changes in Function Derive from Regenerated Connections.8.2.3 Specificity of Regeneration.8.2.4 Heterogeneity of Axon Regenerative Ability.8.2.5 Intraneuronal Mechanisms of Axon Regeneration.8.2.5.1 Cytoskeletal Changes During Axon Retraction.8.2.5.2 Cytoskeletal Changes During Regeneration.8.2.6 Role of Axon-Guidance Molecules in Axon Regeneration in the Spinal Cord.8.2.6.1 Cloning and Sequencing of Axonal-Guidance Molecules from Lamprey CNS.8.2.6.2 Effect of Spinal Transection on Expression of Netrin and its Receptors.8.2.6.3 Expression Patterns of Semaphorins and Their Receptors.8.2.6.4 Effect of Spinal Cord Transection on Semaphorin Expression.8.2.6.5 Evidence from Other Species for Involvement of Guidance Molecules in Axon Regeneration.8.2.6.6 Possible Mechanisms of Involvement of Guidance Molecules in Regeneration.8.3 Conclusions: Where Lampreys Fit Into the Study of Regeneration.References.9 Functional Regeneration in the Larval Zebrafish Spinal Cord (D. Harshad Bhatt, Hana Patzelova, David L. McLean, Joseph R. Fetcho, and Steven J. Zottoli).9.1 Introduction.9.2 Zebrafish Background.9.3 Spinal Cord.9.4 The Escape Circuit.9.5 The Mauthner Neuron.9.5.1 Morphological Characteristics of the Zebrafish M-Cell.9.5.2 Physiological Identification of the M-Cell in Zebrafish.9.6 M-Axon Response to Injury in Adult Teleost Fish and Urodeles.9.6.1 Adult Teleosts and Adult Amphibians.9.7 M-Axon Response to Injury in Larval Amphibians and Larval Zebrafish.9.7.1 Larval Urodeles.9.7.2 Larval Anurans.9.7.3 Larval Zebrafish.9.8 Induction of Regeneration.9.9 Extensions of the Zebrafish Results.References.10 Zebrafish as a Model System for Successful Spinal Cord Regeneration (Catherina G. Becker and Thomas Becker).Overview.10.1 Introduction.10.2 Behavioral Recovery After Spinal Cord Lesion.10.3 Anatomical Basis of Functional Recovery After Spinal Cord Transection.10.3.1 Descending Projections.10.3.1.1 Specificity of Axon Re-Growth from the Brainstem.10.3.2 Ascending Projections.10.4 Environmental Factors Influencing Axon Re-Growth.10.5 Neuron-Intrinsic Changes Associated with Axon Re-Growth.10.5.1 Expression of Growth-Associated Genes.10.5.2 Influence of the Level of Axotomy on the Regenerative Response.10.6 Evidence for Plasticity in the Lesioned Spinal Cord.10.7 Analysis of Spinal Circuitry in Developing Zebrafish.10.8 In-Vivo Approaches to the Study of Regeneration in Zebrafish.10.8.1 Functional Studies of Spinal Cord Regeneration in Adult Zebrafish.10.8.2 Use of Transgenic Fish to Analyze Gene Regulation During Regeneration.10.9 Future Directions.10.10 Conclusions.References.IV Non-Mammalian Models of Visual System Regeneration.11 Functional Aspects of Optic Nerve Regeneration in Non-Mammalian Vertebrates (Sarah A. Dunlop).Overview.11.1 Why the Visual System as a Model for CNS Regeneration?11.1.1 The Four Prerequisites for Visual System Repair.11.1.2 Procedural and Innate Species Variations.11.1.3 Evolutionary Hierarchy of Optic Nerve Regeneration.11.2 Early Behavioral Studies.11.2.1 Behavioral Parameters.11.3 Making a Topographic Map: A Biphasic Process.11.3.1 Molecular Guidance Cues and Restoration of Topography.11.3.2 Neurogenesis and the Restoration of Topography.11.3.3 Spontaneous Activity and the Restoration of Topography.11.3.4 Changing the Balance of Excitation and Inhibition and Restoration of Topography.11.3.5 Modulating Neural Activity to Influence Topography.11.4 Conclusions.References.12 Optic Nerve Regeneration in Goldfish (Satoru Kato, Yoshiki Koriyama, Toru Matsukawa, and Kayo Sugitani).12.1 Introduction.12.2 The Time Course of Optic Nerve Regeneration in Goldfish.12.2.1 Morphological Changes in RGCs and Their Axon Terminals During Optic Nerve Regeneration.12.2.2 A Computer Image Processing System to Quantify Goldfish Behavior.12.3 Time-Specific Molecular Expressions During Optic Nerve Regeneration in Goldfish.12.3.1 Purpurin: A Retinol-Binding Protein in the Retina During the Early Stage of Optic Nerve Regeneration.12.3.2 Molecular Involvement of Na,K-ATPase in the Retina During Axonal Regeneration in Goldfish.12.3.3 Retinal Transglutaminase (TGR) During Axonal Regeneration in Goldfish.12.3.4 Up-Regulation of a Molecule in the Optic Tectum During the Late Stage of Optic Nerve Regeneration.12.4 Applications of Fish-Derived Regeneration-Associated Molecules to Promote Regrowth of Mammalian Optic Axons.12.5 Summary.References.13 Gene Regulation During Axon and Tissue Regeneration in the Retina of Zebrafish (Marie-Claude Senut, Blake Fausett, Matthew Veldman, and Daniel Goldman)13.1 Introduction.13.2 The Zebrafish Retina.13.3 Retina Injury.13.3.1 Experimental Models of Retinal Injury.13.3.2 Gene Regulation in the Injured Zebrafish Retina.13.3.2.1 Phase 1: Programmed Cell Death (Apoptosis) and Immune Response.13.3.2.2 Phase 2: Stem Cell Activation and Proliferation.13.3.2.3 Phase 3: Cellular Migration and Differentiation.13.3.3 Approaches to Identifying Transcriptional Regulators in the Injured Retina: The Example of alpha1-Tubulin.13.4 Optic Nerve Regeneration.13.4.1 Experimental Models of Optic Nerve Regeneration.13.4.2 Gene Regulation Following Optic Nerve Lesion.13.4.2.1 Environmental Factors Influencing Fish Optic Nerve Regeneration.13.4.2.2 Intrinsic Mechanisms of Optic Nerve Regeneration.13.4.3 Approaches to Identifying Transcriptional Regulators of Optic Nerve Regeneration in Zebrafish: The Example of alpha1-Tubulin.13.5 Genetic Strategies for Retinal Regeneration: Perspectives and Challenges.13.6 Concluding Remarks.References.Subject Index.
Verlagsort | Weinheim |
---|---|
Sprache | englisch |
Maße | 170 x 240 mm |
Gewicht | 958 g |
Themenwelt | Medizin / Pharmazie ► Medizinische Fachgebiete |
Naturwissenschaften ► Biologie | |
ISBN-10 | 3-527-61036-7 / 3527610367 |
ISBN-13 | 978-3-527-61036-5 / 9783527610365 |
Zustand | Neuware |
Haben Sie eine Frage zum Produkt? |